Science Blog: Fabulous Tuntsa
Tuntsa is a fabulous wilderness area in eastern Lapland, an uninhabited paradise for all outdoor people. When I was a small boy, I heard a lot of stories at home about Tuntsa, where my father had been during the time of the Second World War. After the war, there were generally two kinds of men: those who never spoke a word about their experiences and those who spoke a lot. My father was something in between; at least every time that the veterans in our village gathered together, someone started to tell war stories, which we young lads listened to very excitedly. My old man often told about his adventures in Tuntsa. I especially remember his commanding officer, Captain Hannila, a hard drinker who my dad appreciated a lot. Anyhow, the unique nature of Tuntsa made such an impression on him that his lifetime dream was once to go back there, but he never managed to do it.
His son had better luck. The Tuntsa area was one of the targets of the Archean project of GTK in the early 2000s, but had not yet been discussed in the project report (Hölttä 2012). However, Tiia Kivisaari from the University of Helsinki wrote her MSc thesis on Tuntsa, in which she described in detail the metamorphism of the area (Kivisaari 2008). Eventually, a paper based on her thesis was published last year in the open access journal Minerals, but also including a lot of new isotopic and mineral analytical data (Hölttä et al. 2020).
Geology
The Tuntsa area (Fig. 1) is considered to be the northwesternmost part of the Archean Belomorian Belt, which is characterized, for example, by eclogites, which are very rare in such old rocks. Eclogites have been found in Russia along and near the White Sea coast, and there has been much discussion about whether the eclogite metamorphism was Archean, Paleoproterozoic or both (Mints & Dokukina 2020). Unfortunately, at least thus far, no eclogites have been found on the Finnish side of the border. Belomorian eclogites were metamorphosed in high, 15–20 kbar pressures, and because eclogites of younger eras are normally related to subductional processes, they are very interesting from the point of view of Precambrian plate tectonics.
![Figure 1. a) Lithological map of the Tuntsa Suite and surrounding areas, simplified from Bedrock of Finland – DigiKP, Digital map database [Electronic resource]. Espoo: Geological Survey of Finland (bedrock_of_finland_200k.xml); b) A pseudocolour magnetic map, purple = magnetic maximum, green = magnetic minimum, the Tuntsa Suite is outlined. In a) the dots with numbers refer to the sites of the studied samples.](https://www.gtk.fi/app/uploads/2021/05/Figure-1-e1621503894756.jpg)
The Tuntsa area comprises typical Neoarchean, 2.7–2.8 Ga granitoid gneisses in the north and south, and between these lies the Tuntsa Suite, which is a polymetamorphic complex mainly consisting of metasedimentary gneisses. The Tuntsa Suite belongs to a long belt of metasedimentary rocks extending hundreds of kilometres to the southeast.
The metamorphic evolution of the area is complicated. Metasedimentary gneisses commonly have medium to coarse-grained staurolite, kyanite and garnet-bearing mineral associations, but similar associations are also found in the Paleoproterozoic rocks west of the Tuntsa Suite. Therefore, the first obvious question is whether the medium-pressure metamorphism was Proterozoic or Archean. The Suite is characterized by subhorizontal foliation, which obliterates earlier structures and also earlier migmatisation. The abundance of staurolite in rocks with migmatitic structures is a problem, because in most whole-rock compositions, staurolite is only stable in subsolidus pressure–temperature conditions Staurolite-bearing rocks should not therefore show signs of partial melting, unless staurolite is a retrograde mineral which is evidently not the case in Tuntsa where euhedral, centimetre-sized crystals are common.
Age determinations
To determine the temporal evolution of the Tuntsa metasediments, we first dated zircon grains from a metasedimentary gneiss sample. Zircon was expected to be at least partly detrital, in which case the deposition of the sediment must have been younger than the age of the youngest grains. Zircon was dated using the Nordsim ion microprobe in Stockholm and the laser ablation mass spectrometer (LA-ICP-MS) at GTK, Espoo. The Nordsim data revealed two age populations, the older being 2.77–2.84 Ga and the younger being 2.65–2.70 Ga. The LA-ICP-MS data displayed an almost continuous spread of ages from 2.75–2.55 Ga, with most dates being younger than 2.70 Ga.
The abundance of 2.65–2.70 Ga zircon grains might indicate that the deposition of the Tuntsa sediments was younger than this. However, many zircons of that age have low Th/U ratios that are typical for metamorphic grains. Also, during that period, a strong metamorphic and melting event occurred all over the present Fennoscandian Shield. Therefore, it is more probable that these zircons are metamorphic rather than detrital. We also dated an igneous gneissose trondhjemite located within the paragneisses. This yielded two zircon populations of 2.85 Ga and 2.76 Ga. The latter was interpreted to represent the age of the intrusion, in which case the sedimentation would have been older than 2.76 Ga. However, the contacts of this intrusion are not exposed, and elsewhere in the Suite the contacts of similar intrusive rocks with the paragneisses appear to be tectonic rather than intrusive. Therefore, the age of the trondhjemite does not necessarily constrain the deposition age of the metasediments.
Other datings yielded Paleoproterozoic ages. Monazite was directly dated from thin sections of five samples from metasedimentary rocks. No Archean ages were found, all the monazites giving ages of 1.79–1.80 Ga. Garnet was dated from one sample using the Sm–Nd method, and the garnet-whole rock date was also around 1.8 Ga. Tourmaline-bearing pegmatites are common in the Tuntsa Suite. The LA-ICP-MS dating on zircon from a pegmatite yielded both Archean and Paleoproterozoic, 1.89–1.92 Ga dates, the youngest overgrowth rim on an Archean zircon yielding an age of 1.82 Ga.
Mineral analytics
The mineral analytics also revealed interesting findings. Almost all garnet grains were strongly zoned, indicating changing pressure and temperature conditions during their growth. Apart from the electron microprobe, the mineralogy of the selected samples was analysed by automated scanning electron microscopy with energy dispersive spectrometers (SEM-EDS), using a QEMSCAN® 4300, at the Camborne School of Mines, University of Exeter, UK. The QEMSCAN analyses revealed spectacular zoning in some garnets with an almost perfect euhedral core overgrown by an outer rim whose composition differs considerably from the core (Fig. 2).
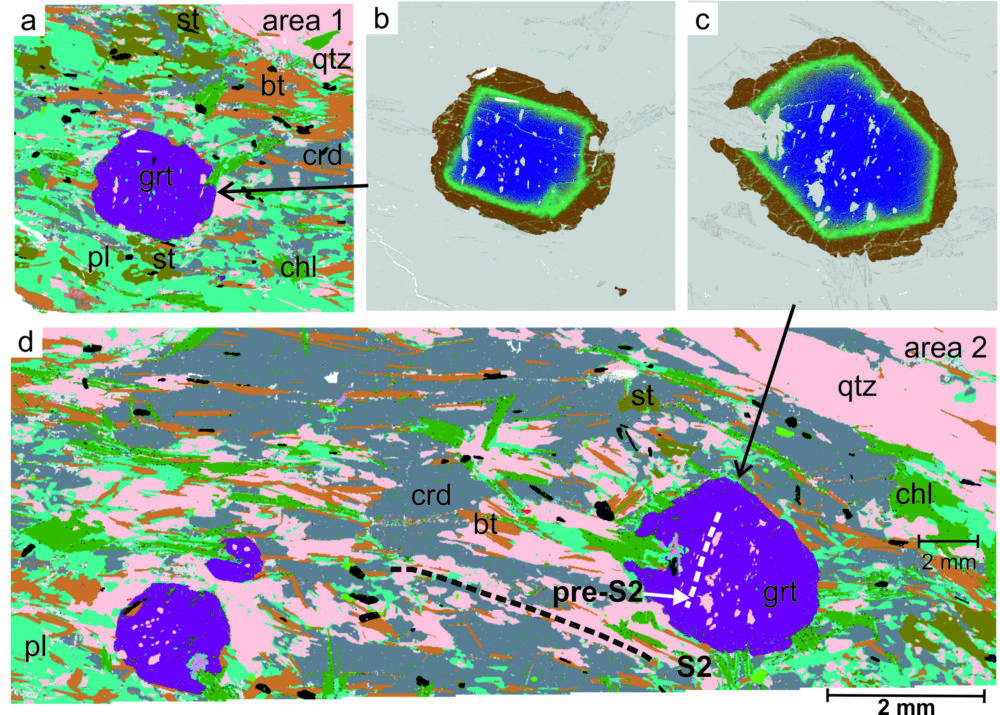
Pressure–temperature–time evolution
The pressure–temperature evolution of the Tuntsa paragneisses was modelled using Perple_X thermodynamic software. This produces, for example, PT pseudosections that are useful in interpreting metamorphic mineral assemblages and compositional zoning in minerals. The garnets displayed in Figure 2 have a Ca-rich, Mg-poor core that was crystallized at c. 530–540 °C and 6 kbar, corresponding to crustal depths of c. 20 km. However, garnet–staurolite–kyanite–biotite assemblages are common in the Tuntsa Suite, and according to Figure 3, these should be stable at much higher temperatures and pressures, at around 630–680 °C and 8–10 kbar, provided that H2O was in excess. This means that the garnet core started to crystallize much earlier than staurolite and kyanite, and garnet is not in chemical equilibrium with the latter minerals. The outer rims of garnet presented in Figure 2 are Ca-poor but Mg-richer than the cores, suggesting that the rims crystallized at lower pressures and higher temperatures than the cores.
Based on mineral compositions and textures, the rocks followed a PT path where the pressure and temperature increased from ca. 6 kbar and 530 °C to 9 kbar and 650 °C. This was followed by cooling and decompression down to ca. 550 ℃ and even 2–3 kbar, indicated by andalusite crystallization and cordierite formed in the breakdown of staurolite and biotite + kyanite. However, if the system is H2O-undersaturated it expands considerably the PT-field where grt-ky-st assemblage is stable so that these minerals can coexist also in temperatures much below c. 650 ℃. The H2O-undersaturated system may the case in polymetamorphic rocks such as those in Tuntsa, where the earlier high-grade metamorphism and partial melting already consumed a lot of the hydrous phases whose decomposition is needed to keep the system H2O-saturated. Discussion about this issue was not included in our paper which is one of the deficiencies of the study. Based on the age determinations on monazite and garnet, we think that in any case this PT evolution took place during the Paleoproterozoic.
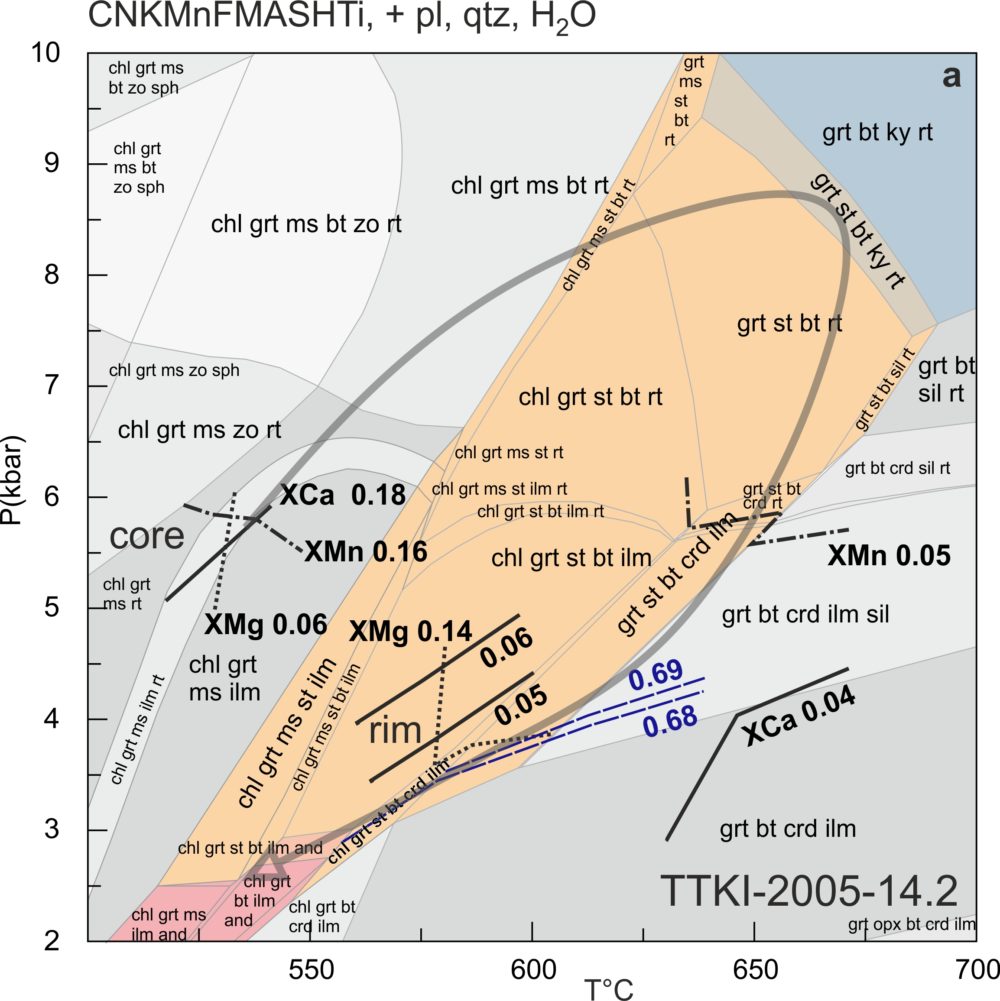
The observed garnet zoning where Mg increases and Ca decreases from the core to the rim was also problematic. In general, in pelitic whole-rock compositions, the Ca content of garnet decreases with decreasing pressure if the bulk composition is fixed. However, similar zoning, although not as abrupt as in Figure 2, was also developed in cordierite-free garnet–kyanite–staurolite–biotite–plagioclase–quartz assemblages that did not show any textural evidence of decompression. The reason for this is presumably the fact that garnet and plagioclase cores have sequestered the available Ca in the rock. If the Ca content is low in the effective whole-rock composition, which also controls the garnet composition, the low-Ca garnet rims can develop with increasing pressure and temperature.
The Tuntsa Suite represents a polymetamorphic area that underwent several tectonic and metamorphic events over a time scale of nearly 1 billion years. The first high-grade metamorphism was in the Neoarchean at around 2.70‒2.64 Ga, indicated by the remnants of migmatisation and U–Pb dates of metamorphic zircon. During the Paleoproterozoic, metasedimentary gneisses were again penetratively deformed and strongly recrystallized under medium pressures, producing staurolite, kyanite and garnet-bearing mineral assemblages. This was presumably related with the tectonic thickening of the crust at around 1.89 Ga, related with the overthrusting of the Lapland granulite complex onto the adjacent rocks in the south. After this, the rocks started to exhume, and the final low-pressure metamorphism took place at ca. 1.79 Ga, suggested by the monazite datings.
Epilogue
My father did not have much chance to travel. The only vehicles he owned were a bicycle, a kick sled and a horse and carriage, and this, combined with a perpetual shortage of money, did not allow him to realize his dream journey to Tuntsa from southern Savo, where our home was. During his lifetime, he only made two trips to Helsinki and one longer trip abroad, to the Soviet Union. Fortunately, the government paid for the latter, but on the other hand, it was not all the time so pleasant; he had to walk much of the journey, the accommodation was poor, and from time to time there was too much lead in the air to make life enjoyable. My dad was, however, a wise guy, or at least clever enough to send me to the secondary school admission test. This was in the summer of ’65, when the Beatles staged their second concert tour of the United States and the world started to change. I passed the test and started my higher education, which is still ongoing. And as a result of my parents’ decision, I ended up in Tuntsa during the course of my career. The old man would have been happy for my sake.
References
Hölttä, P. 2012 (ed.). The Archaean of the Karelia Province in Finland. Geological Survey of Finland, Special Paper 54. 254 p. Available at: https://tupa.gtk.fi/julkaisu/specialpaper/sp_054.pdf
Hölttä, P., Kivisaari, T., Huhma, H., Rollinson, G., Kurhila, M. & Butcher, A. R. 2020. Paleoproterozoic Metamorphism of the Archean Tuntsa Suite, Northern Fennoscandian Shield. Minerals 10 (11), 1034.
Kivisaari, T. 2008. Tuntsan metasedimenttivyöhykkeen metamorfoosi. MSc Thesis, Department of Geosciences and Geography, University of Helsinki. 52 p.
Mints, M. V. & Dokukina, K. A. 2020. Age of eclogites formed by the subduction of the Mesoarchaean oceanic crust (Salma, Belomorian Eclogite Province, eastern Fennoscandian Shield, Russia): A synthesis. Precambrian Research 350.
Text: Pentti Hölttä
Dr Pentti Hölttä has worked as a bedrock geologist and senior scientist at the Geological Survey of Finland (GTK) since August 1984. He has also been an adjunct professor at the University of Helsinki since the 2003, teaching metamorphic petrology to undergraduate students and supervising MSc and PhD theses. His main research interests have been in metamorphic and Archean geology. During recent years, Pentti Hölttä has compiled a metamorphic map of Finland and applied U–Pb dating of monazite to unravel the temporal evolution of metamorphism in the Svecofennian orogen.